The focus of our research program is the structure determination of integral membrane proteins by X-ray crystallography and functional analysis of these proteins using biophysical, biochemical, and cell biological techniques.
- We study transporters embedded in the outer membranes of Gram-negative bacteria, which are surface accessible and therefore have the potential to be good vaccine and/or chemotherapeutic targets against infectious diseases. We also study the membrane associated or soluble protein partners that interact with outer membrane transporters to better understand how these systems function in vivo. Current topics in the lab include:
-
- small molecule and protein import across the bacterial outer membrane,
- outer membrane protein biogenesis, and
- protein import across mitochondrial outer membranes.
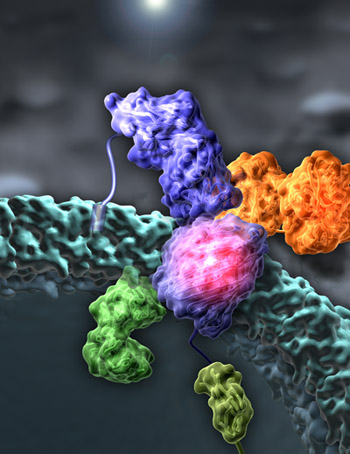
Metal transport mediated by transporters located in the outer membrane of gram-negative bacteria is important for bacterial growth. These transporters are accessible, and thus attractive vaccine targets against pathogens such as Neisseria, Haemophilus, and Yersinia. Unanswered questions in the field include how substances are individually recognized by dedicated transporters, how a signal is transduced across the outer membrane to initiate the transport process, and how protein toxins use these small molecule transporters to enter and kill a cell. Recent results are described below:
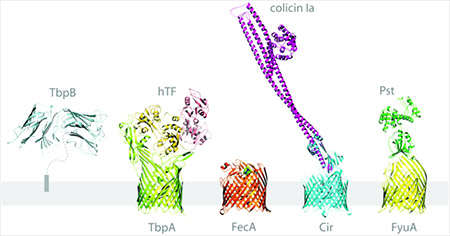
Our structures of Yersinia pestis FyuA and pesticin allowed us to engineer a novel phage therapy drug against a Gram-negative pathogen. To understand how toxin is transported across the outer membrane, we solved the structure of FyuA, a TonB-dependent iron transporter required for virulence in bubonic plague, with and without its cognate siderophore, ferric yersiniabactin. We also determined the structure of pesticin, a bacterial toxin that uses FyuA to cross the outer membrane. Our structural studies showed that the ‘killing’ domain of pesticin resembles phage T4 lysozyme. We engineered a hybrid bacterial-phage toxin containing a FyuA bacterial targeting domain and a phage killing domain. We showed that the hybrid lysin evades the natural protection mechanism of toxin-producing strains and kills all Yersinia strains tested in a mouse model of pneumonic plague. This is the first demonstration of phage therapy for Gram-negative pathogens. We are currently using the technology to develop phage therapy tools for other bacterial pathogens, such as Klebsiella pneumoniae.
The crystal structure of TbpA bound to human transferrin reveals the mechanism of iron piracy by pathogenic Neisseria. Pathogenic Neisseria are responsible for bacterial meningitis, meningococcal septicaemia, and gonorrhoea. They use the largest known TonB-dependent iron transporter (TbpA, 100 kDa) to acquire iron from human transferrin, an 80 kDa glycoprotein that circulates in the bloodstream. Recognition of iron-bound transferrin is facilitated by TbpB, a 75 kDa soluble protein attached to the outside of the outer membrane by a lipid anchor. Nesseria are obligate human pathogens and can only import iron from human proteins like transferrin; TbpA and TbpB are required for virulence and are present in all clinical isolates, making them important therapeutic targets. To better understand how two bacterial proteins specifically bind a human protein then extract and transport iron into the periplasm, we solved the structures of TbpA in the presence and absence of human transferrin. This is one of the largest outer membrane protein complexes characterized at high resolution and has direct translational applications. We identified key residues and surface features important for vaccine and drug development.
Acinetobater baumannii contains zinc-regulated TonB-dependent transporters that increase virulence. A. baumannii is a nosocomial pathogen that has developed multidrug resistance and therefore is a major health concern. Although many Gram-negative bacteria import zinc through non-specific pathways, A. baumannii encodes three TonB-dependent metal transporters that are regulated by zinc levels. We are currently assessing the structures and functions of these transporters, their function in the cell, and their role in A. baumannii virulence. This work could lead to novel drugs against this highly-resistant pathogen.
Gram-negative bacteria, mitochondria, and chloroplasts contain an inner and outer membrane. The outer membrane contains a host of beta barrel proteins commonly called outer membrane proteins (OMPs), which serve essential functions in cargo transport and signaling and are also vital for membrane biogenesis. In Gram-negative bacteria, it is known that OMPs are synthesized in the cytoplasm and then transported across the inner membrane into the periplasm via a Sec translocon.
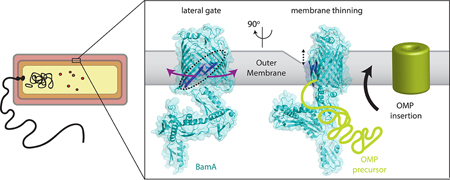
Once in the periplasm, chaperones guide the nascent OMPs across the periplasm and peptidoglycan to the inner surface of the outer membrane. Here, the nascent OMPs are recognized by a complex known as the beta barrel assembly machinery (BAM) complex which folds and inserts the new OMPs into the outer membrane.
We solved structures of BamA which contains a large N-terminal periplasmic domain and a C-terminal 16-stranded β-barrel domain. The periplasmic domain was found in two different conformations representing open and closed states, which may serve as a gating mechanism to allow substrate access to the internal barrel cavity. Interestingly, the closed state was accompanied by a significant destabilization of the terminal beta strand, which was found tucked inside the barrel domain. MD simulations revealed that BamA could destabilize the local membrane along the terminal strand, thinning the membrane by as much as 16 Angstroms. In addition, these MD simulations also revealed that the barrel domain of BamA can undergo a lateral opening to create a portal from the periplasm directly into the outer membrane. This work was published in Nature in 2013, with follow-up experiments confirming that lateral opening of the beta barrel is required for BAM function published in Structure, 2014. We are currently investigating the potential of BamA to serve as a drug target for the development of novel antibiotics, since it is an essential protein in all Gram-negative bacteria.
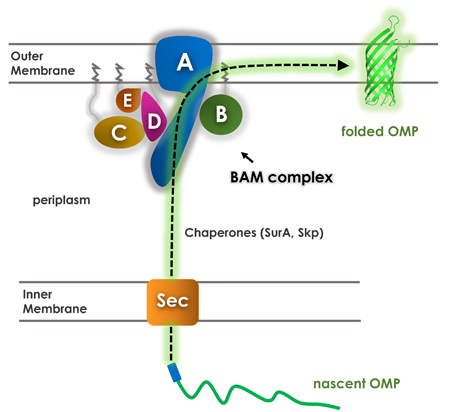
The only other outer membrane protein essential in most Gram-negative bacteria is a protein complex called LptDE, which inserts newly-synthesized lipopolysaccharide (LPS) into the the outer membrane. We recently solved the structures of three LptDE homologs from three distinct bacterial pathogens and are currently assessing their structures and functions. This family of proteins is another excellent target for the development of new antibiotics.
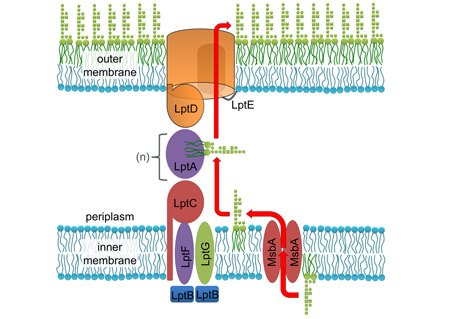
A natural and challenging extension of our work on bacterial transporters is to initiate structural projects on mitochondrial outer membrane transporters. In humans, almost all of the 1000 - 1500 proteins that reside in mitochondria are nuclear-encoded and thus synthesized in the cytoplasm, so they must be imported through dedicated protein import channels. Like Gram-negative bacteria, mitochondria contain two membranes separated by an inter-membrane space. In the mitochondrial outer membrane, the universal protein import channel is Tom40, part of the TOM (translocase of the outer membrane) and TIM (translocase of the inner membrane) transport system. Tom40 is predicted to be a β-barrel import channel, similar to bacterial outer membrane transporters.
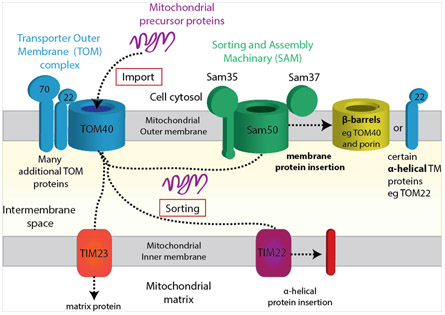
From a structural perspective, the TOM and SAM complexes illustrate the future challenges faced by the field: we want to understand large, multi-protein, human membrane protein complexes through structural and functional characterization. In addition to Tom40, mitochondria make Sam50, a homolog of BamA (discussed under biogenesis of bacterial outer membrane proteins) essential for biogenesis of mitochondrial outer membrane proteins. A third predicted mitochondrial outer membrane protein is Mdm10, which interacts with Sam50 and also is involved in tethering mitochondria to the endoplasmic reticulum for lipid transport between the two organelles. Determining structures for Tom40, Sam50, Mdm10, and their associated complexes will give us a better understanding of mitochondrial protein biogenesis, import, and transport. On-going projects in the lab address all three of these topics.
Klebsiella pneumoniae capsules play a variety of roles in pathogenesis, including helping to shield the bacteria from the immune system and possibly protecting it in other environments. Regulation of capsule synthesis is under the control of a signaling cascade that is conserved in other enterobacteria and was first described and studied at the NIH by S. Gottesman (NCI). We are investigating the possibility of targeting the capsule regulators to help control Klebsiella infections, by using a combination of genetic approaches and structural approaches to further define the capsule signaling cascade in Klebsiella. In parallel, we are carrying out small molecule screens for compounds that perturb regulation of capsule synthesis. This work should provide a much better understanding of the regulation of capsule synthesis in Klebsiella, possible lead compounds for development of novel antibiotics aimed at changing bacterial behavior rather than killing the bacteria, and provide structural insight into some of the unique aspects of the capsule regulatory cascade, relevant to a broad group of Gram-negative bacteria.
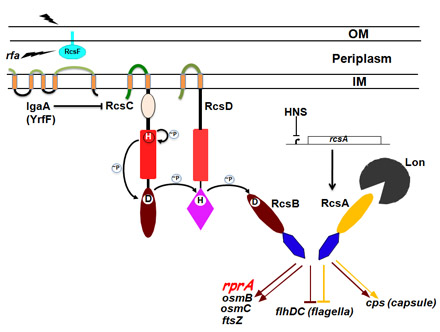
The capsules of Klebsiella are under the control of the Rcs regulatory cascade, first identified and studied extensively in the Gottesman lab in E. coli. All the major regulatory elements are conserved in Klebsiella, with >75% identity with the proteins found in E. coli. Mutations in the RcsB positive regulator for this cascade totally block capsule synthesis. Null mutations in a negative regulator (named IgaA in Salmonella) are lethal in both Salmonella and E. coli, apparently because a product or products activated by the Rcs system are lethal. IgaA is a largely unstudied integral membrane protein that has no structural homologs, so we initiated a structural investigation of this protein with the long-term goal to understand its role in regulation of capsule synthesis, including interacting partner(s) and mechanism of action. We have expressed and purified IgaA from K. pneumoniae and other bacterial homologs and are currently establishing crystallization conditions for this novel membrane protein.