Our research focuses on visualizing and understanding ribonucleic acids, or RNAs, that
aren't used as templates to make proteins. RNAs are polymers similar to DNA but
subtle chemical differences of their composition allow them to fold into elaborate three dimensional
objects and nanomachines, beyond the double-helix shape that DNA form.
Complex structures formed by RNA are capable of recognizing and interacting with
other molecules in the cell, large and small, with extraordinary affinity and exquisite
selectivity, and can even catalyze many chemical reactions. Some viruses are made of
RNA, or temporarily take the form of RNA in the cell during their life cycle. We are
working to uncover the general principles of how RNA structures are built and
organized, how RNA structures move and change their shape, and how RNAs interact
with proteins and other RNAs in the cell. Such fundamental understanding of RNA will
allow new therapeutics to be developed to modulate these biologically important
molecules.
- Current Research Topics include:
- Structures and mechanisms of gene-regulatory riboswitches.
- tRNA-mediated stress response pathways in eukaryotes
- Host and viral noncoding RNA structures that control immune protein activities.
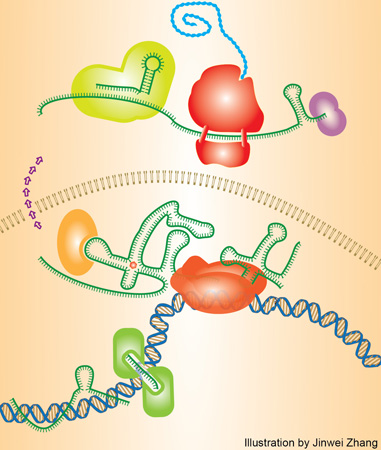
Structured noncoding RNAs play crucial roles in numerous cellular processes
Riboswitches are non-coding RNA elements that directly recognize intracellular metabolites using their elaborate tertiary structures and modulate transcription, translation, splicing, etc., through ligand binding-induced conformational switching. The widespread bacterial T-box riboswitches are a fascinating class of structured mRNAs that recognize specific tRNAs as their ligands. Remarkably, the T-boxes directly sense the aminoacylation states of bound tRNAs to gauge intracellular amino acid availability, and direct a transcriptional or translational switch to regulate amino acid biosynthesis, transport, and tRNA aminoacylation.
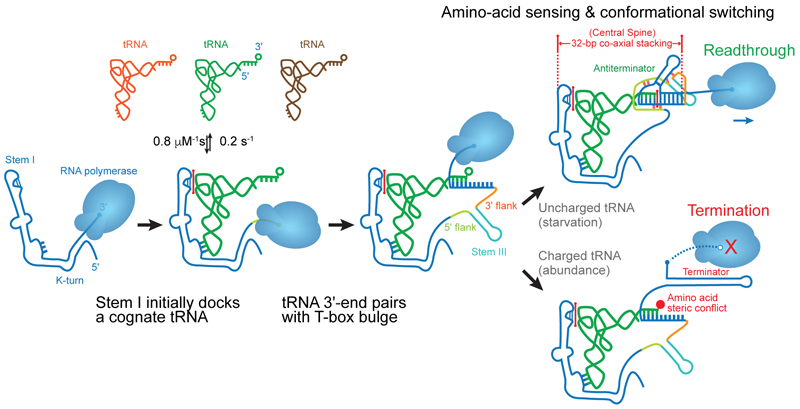
Proposed mechanism of a transcriptionally acting T-box riboswitch
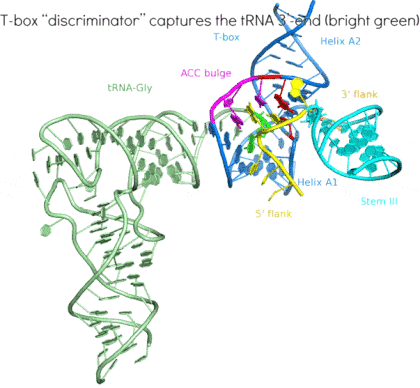
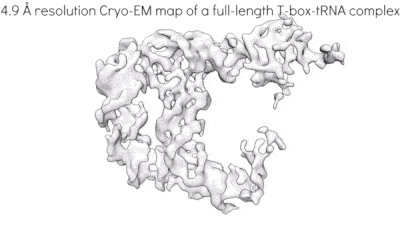
Li, Su, et al., Chiu, and Zhang, 2019, Nat. Struct. & Mol. Biol.
In addition to the transcription-regulating T-boxes illustrated above, new T-boxes have recently been identified that regulate translation initiation. These translational T-boxes tend to have a much shorter Stem I and require a intervening Stem II domain absent from the glycine-specific T-boxes above. Unexpectedly, the Stem II domain acts to locally reinforce the codon-anticodon interactions between Stem I and tRNA. Together, Stems I and II dock to form a RNA pincer to capture the anticodon of the tRNA.
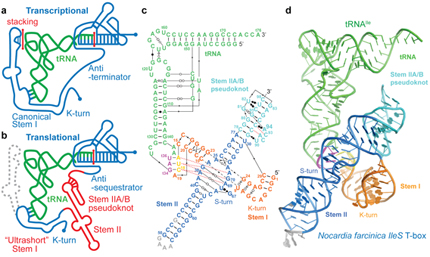
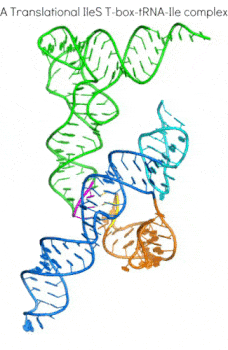
In addition to their canonical adaptor roles in protein synthesis, throughout evolution tRNAs have acquired numerous non-canonical roles in gene regulation and metabolic control. It is increasingly clear that the aminoacylation state of tRNAs can serve as crucial indicators of overall cellular well-being in both bacteria and eukaryotes. In stressed eukaryotic cells, rapidly deacylated tRNAs bind to and activate the Gcn2 kinase, the founding member of the eIF2alpha kinases, leading to global translation suppression and metabolic reprogramming to prioritize survival over growth. We are working to uncover the structural basis and molecular mechanisms by which deacylated tRNAs activate the crucial kinase activity of Gcn2.
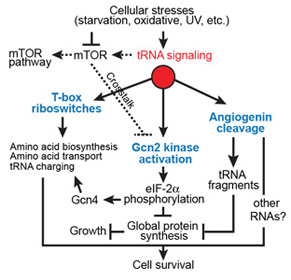
tRNA-mediated stress response pathways.T-boxes (in bacteria) and Gcn2 (in eukaryotes) recognize uncharged tRNAs as cellular signals of stress, reprogram cellular metabolism to prioritize survival over growth. Human angiogenin additionally signals stress by cleaving tRNAs and likely other RNAs.
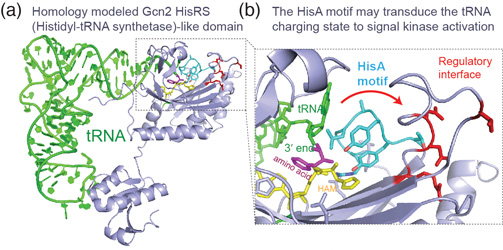
Stress signaling in eukaryotes via the tRNA-activated Gcn2 kinase
Highly structured viral RNA elements are adept at manipulating and subverting host protein activities for their own benefit using mimicry and subterfuge. An exemplary case is the differential regulation of human antiviral kinase Protein Kinase RNA-activated (PKR) by diverse structured RNAs from viruses and the host. By determining their 3D structures and mapping their interactions, we are working to define the hallmarks of RNA activators and inhibitors of the same enzyme. These work will illuminate how structured RNAs control protein activities.